Gene Expression Introduction
Gene Expression: Genes serve as the fundamental units of inheritance, carrying genetic information that dictates the traits of an organism. The instructions within genes guide the synthesis of proteins, which are crucial for various cellular functions. Here’s an overview of gene expression:
- Genetic Information in Genes: Genes contain the genetic information necessary to determine specific traits in an organism. They are composed of DNA sequences that encode instructions for building proteins.
- Protein Functions: Proteins are essential molecules in cells, contributing to the structure, function, and regulation of biological processes. They perform diverse roles, including enzymatic activities, structural support, signaling, and transport.
- Gene Activation and Protein Synthesis: The process of turning on genes to produce proteins is known as gene expression. Gene activation results in the synthesis of messenger RNA (mRNA), which serves as a template for protein production. The flow of genetic information, from gene to mRNA to protein, is the essence of gene expression.
- Activation Timing and Protein Functions: For a cell to function effectively, it is crucial that specific proteins are produced at the right time and in the right quantities. Gene expression ensures the timely and regulated creation of proteins, allowing cells to perform their functions.
- Messenger RNA (mRNA): During gene expression, the information encoded in DNA is transcribed into mRNA. This mRNA molecule carries the genetic code from the nucleus to the cytoplasm, where protein synthesis takes place.
- Importance of Gene Expression: Gene expression is a vital process that underlies cellular activities. It governs the production of proteins that are involved in growth, development, response to environmental signals, and the maintenance of cellular homeostasis.
- Regulation of Gene Expression: Cells tightly regulate gene expression to respond to changing conditions. Factors such as environmental cues, developmental stages, and cellular needs influence when and how genes are activated.
- Cellular Function: Proper gene expression is essential for the normal functioning of cells. Aberrations in gene expression can lead to various diseases, developmental disorders, and cellular dysfunctions.
In summary, gene expression is a dynamic and tightly regulated process that ensures the accurate synthesis of proteins required for cellular functions. It plays a central role in the complex orchestration of biological processes within living organisms.
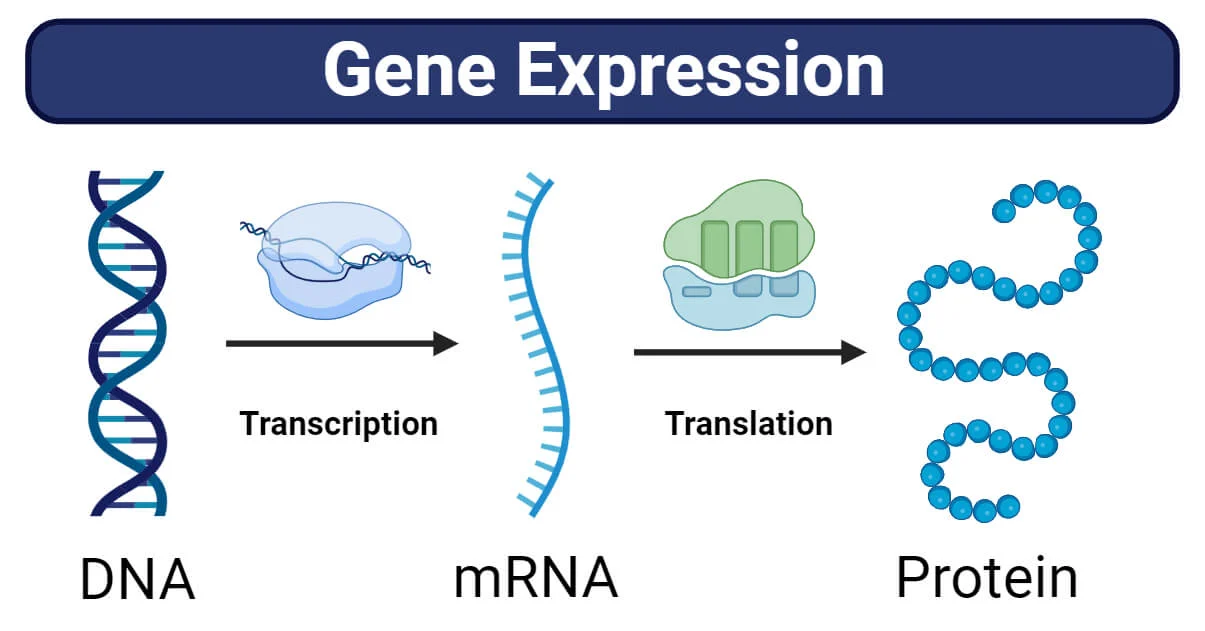
Steps in Protein Synthesis: Transcription and Translation
- Gene Activation:
- When a cell needs a specific protein, the corresponding gene is activated.
- Gene activation is often regulated by various cellular signals, environmental cues, or developmental stages.
- Transcription:
- The activated gene undergoes transcription, a process that occurs in the cell nucleus.
- During transcription, an enzyme called RNA polymerase reads the DNA sequence of the gene and synthesizes a complementary messenger RNA (mRNA) molecule.
- The mRNA serves as a copy of the gene and carries the genetic information from the nucleus to the cytoplasm.
- mRNA Processing:
- Before leaving the nucleus, the newly synthesized mRNA may undergo processing, including the addition of a 5′ cap and a poly-A tail, as well as the removal of introns (non-coding regions).
- These modifications enhance the stability and functionality of the mRNA.
- mRNA Export to Cytoplasm:
- The processed mRNA is transported from the nucleus to the cytoplasm, where protein synthesis takes place.
- Translation:
- Translation occurs in the cytoplasm at ribosomes, cellular structures composed of proteins and RNA.
- During translation, the information carried by the mRNA is read in triplets called codons.
- Each codon corresponds to a specific amino acid, and the sequence of codons determines the sequence of amino acids in the protein.
- Amino Acid Assembly:
- Transfer RNA (tRNA) molecules bring individual amino acids to the ribosome, guided by the mRNA codons.
- The ribosome facilitates the assembly of amino acids in the correct order, forming a polypeptide chain.
- Protein Folding and Function:
- The newly synthesized polypeptide undergoes folding to attain its three-dimensional structure.
- The specific sequence of amino acids determines the protein’s structure and, consequently, its function within the cell.
- Post-Translational Modifications:
- After synthesis, proteins may undergo post-translational modifications, such as phosphorylation or glycosylation, which further modify their structure and function.
- Cellular Functions:
- The synthesized protein carries out specific functions within the cell, contributing to cellular processes, signaling pathways, and maintaining cell homeostasis.
In summary, the process of protein synthesis involves the activation of a gene, transcription to produce mRNA, and translation to assemble amino acids into a functional protein. This intricate process ensures the accurate production of proteins required for various cellular functions.
Stages in Gene Expression
Gene expression is a dynamic process that orchestrates the flow of genetic information from DNA to functional proteins. Understanding these stages provides insights into cellular functions, development, and the molecular basis of diseases. The intricacies of gene expression regulation contribute to the complexity and diversity of living organisms.
Transcription: Decoding Genetic Information
Definition: Transcription is a pivotal process in gene expression, converting the genetic information stored in DNA into a functional form – messenger RNA (mRNA). This essential step sets the stage for subsequent protein synthesis.

Key Steps in Transcription:
- Initiation:
- RNA polymerase binds to a specific DNA region known as the promoter.
- The promoter acts as a signal, marking the starting point for transcription.
- Unwinding DNA:
- RNA polymerase unwinds the DNA double helix near the promoter.
- This exposes a single DNA strand that serves as a template for mRNA synthesis.
- Elongation:
- RNA polymerase moves along the DNA template, adding complementary RNA nucleotides to the growing mRNA chain.
- Synthesis occurs in the 5′ to 3′ direction, mirroring the DNA template.
- Termination:
- Transcription continues until a termination signal in the DNA sequence is reached.
- This signal prompts RNA polymerase to detach from the DNA, releasing the newly formed mRNA molecule.
Additional Aspects in Eukaryotes:
- RNA Processing:
- In eukaryotes, the initial RNA transcript (pre-mRNA) undergoes modifications in the nucleus.
- Modifications include capping (adding a protective cap), splicing (removing non-coding regions), and adding a poly-A tail (stabilizing the mRNA).
- Compartmentalization:
- In prokaryotes, transcription and translation occur in the same cellular compartment.
- Eukaryotes exhibit compartmentalization; transcription occurs in the nucleus, and mature mRNA is transported to the cytoplasm for translation.
Significance: Transcription plays a crucial role in gene regulation and protein synthesis. Understanding this process provides insights into how genetic information is harnessed to build the molecules that govern cellular functions.
Connection to Translation: The transcribed mRNA serves as the template for translation, the subsequent step in gene expression where amino acids are assembled into proteins based on the mRNA sequence. Together, transcription and translation orchestrate the flow of genetic information from DNA to functional proteins in living organisms.
Translation: Building Proteins from mRNA Instructions
Definition: Translation is a fundamental cellular process that transforms the information encoded in mRNA into a functional protein. This intricate mechanism is vital for synthesizing the diverse array of proteins necessary for cellular structure and function.

Key Steps in Translation:
- Initiation:
- Translation commences when mRNA binds to a ribosome, which recognizes the start codon (AUG) on the mRNA.
- The start codon marks the initiation point for protein synthesis.
- tRNA Matching:
- Transfer RNA (tRNA) molecules, each carrying a specific amino acid, align with their anticodon sequences complementary to the mRNA codons.
- This matching ensures the correct amino acids are brought in sequence for protein assembly.
- Elongation:
- The ribosome traverses along the mRNA during elongation.
- Each mRNA codon is paired with the corresponding tRNA anticodon, leading to the sequential addition of amino acids.
- This process forms a polypeptide chain, constituting the primary structure of the protein.
- Termination:
- Translation persists until a stop codon (UAA, UAG, or UGA) is encountered on the mRNA.
- Stop codons signal the termination of protein synthesis.
Post-Translation Modifications: After translation, the synthesized protein may undergo post-translational modifications:
- Folding: Attainment of a specific three-dimensional shape.
- Functional Group Addition: Addition of chemical groups to enhance functionality.
- Cleavage: Removal of specific segments to activate the protein.
Significance: Translation plays a pivotal role in gene expression, translating the genetic code into functional proteins. This process is tightly regulated, contributing to the diversity and specificity of proteins essential for cellular processes.
Connection to Transcription: Transcription and translation are interconnected processes in the central dogma of molecular biology. Transcription produces mRNA, serving as the template for translation. Together, these processes ensure the accurate transfer of genetic information from DNA to functional proteins.
Regulation of Gene Expression: Orchestrating Cellular Functions
Introduction: Regulation of gene expression is a finely tuned process essential for orchestrating when and how genes produce proteins. This dynamic control ensures that proteins are synthesized in a timely and cell-specific manner, contributing to the complexity and functionality of living organisms.

Stages of Gene Expression Regulation:
- Transcriptional Regulation:
- Mechanism: Involves interactions at specific DNA sites and epigenetic effects.
- Key Players:
- Transcription Factors: Interact with DNA, enhancing or inhibiting RNA polymerase binding.
- Epigenetic Modifications: Alter gene accessibility without changing DNA sequence (e.g., DNA methylation, histone alterations).
- Outcome: Modulates the initiation and rate of transcription.
- Post-Transcriptional Regulation:
- Mechanism: Involves modifications to transcribed mRNA.
- Key Processes:
- Splicing: Removal of non-coding introns and joining of protein-coding exons.
- mRNA Processing: Modifications to protect mRNA ends from degradation.
- Outcome: Influences mRNA stability and structure.
- Translational Regulation:
- Mechanism: Controls the initiation and progression of translation.
- Regulatory Factors:
- MicroRNAs (miRNAs): Bind to mRNA, blocking translation initiation or causing mRNA degradation.
- Translational Repressors: Interfere with translation initiation.
- RNA-Binding Proteins: Influence translation through binding to specific mRNA sequences.
- Outcome: Modulates protein synthesis levels.
- Post-Translational Regulation:
- Mechanism: Modifies structure and function of translated polypeptides.
- Common Modifications:
- Phosphorylation: Addition or removal of phosphate groups.
- Acetylation: Addition or removal of acetyl groups.
- Outcome: Alters protein activity, stability, and functional properties.
Significance: Regulation of gene expression is crucial for cellular homeostasis, development, and response to environmental cues. Dysregulation can lead to diseases, emphasizing the importance of understanding and controlling these regulatory mechanisms.
Interconnected Nature: These regulatory stages are interconnected, forming a sophisticated network that allows cells to adapt to changing conditions, ensuring proper gene expression for diverse cellular functions. Understanding this complexity provides insights into cellular behavior and potential therapeutic interventions.
Methods for Measuring Gene Expression: Unraveling Cellular Dynamics
1. Northern Blotting:
- Principle: Hybridization-based method for RNA size and quantity determination.
- Procedure:
- Separation of RNA by gel electrophoresis.
- Transfer to a nitrocellulose membrane.
- Hybridization with labeled probes.
- Pros: Simple, cost-effective.
- Cons: Time-consuming, low throughput.
2. Quantitative Polymerase Chain Reaction (qPCR):
- Principle: Real-time measurement of gene expression through cDNA amplification.
- Procedure:
- Conversion of mRNA to cDNA.
- Amplification using PCR with fluorescent labels.
- Real-time fluorescence measurement.
- Pros: Easy, relatively short assay time.
- Cons: Requires prior target sequence knowledge, limited throughput.
3. Microarray:
- Principle: Hybridization-based measurement of gene expression using cDNA strands.
- Procedure:
- Oligonucleotide/cDNA hybridization on a chip.
- High-throughput analysis.
- Pros: High-throughput, simultaneous analysis of multiple transcripts.
- Cons: Separate preparation of control and test samples, specialized equipment needed.
4. RNA-Seq:
- Principle: Direct sequencing of RNA for gene expression measurement.
- Procedure:
- RNA sample processing into libraries.
- Sequencing and computational analysis.
- Pros: Higher range, detection of various RNA types.
- Cons: Higher cost, increased computational resources.
Significance: These methods play a pivotal role in understanding cellular processes, enabling researchers to unravel the intricacies of gene expression dynamics. The choice of method depends on factors like throughput, accuracy, and the specific biological question at hand.