The semiconservative model of DNA replication , proposed by James Watson and Francis Crick, describes the process where two copies of the original DNA molecule are produced. In this model:
- The original DNA molecule with its two complementary strands serves as a template.
- Each original strand separates, and new nucleotides are added to the exposed bases.
- The end result is two DNA molecules, each consisting of one original strand paired with one newly synthesized strand.
This process ensures that genetic information is faithfully passed on to daughter cells during cell division. The term “semiconservative” reflects the conservation of half of the original DNA in each newly synthesized molecule.
Comprehensive overview
Comprehensive overview of the process of DNA replication based on Watson and Crick’s double helix model.
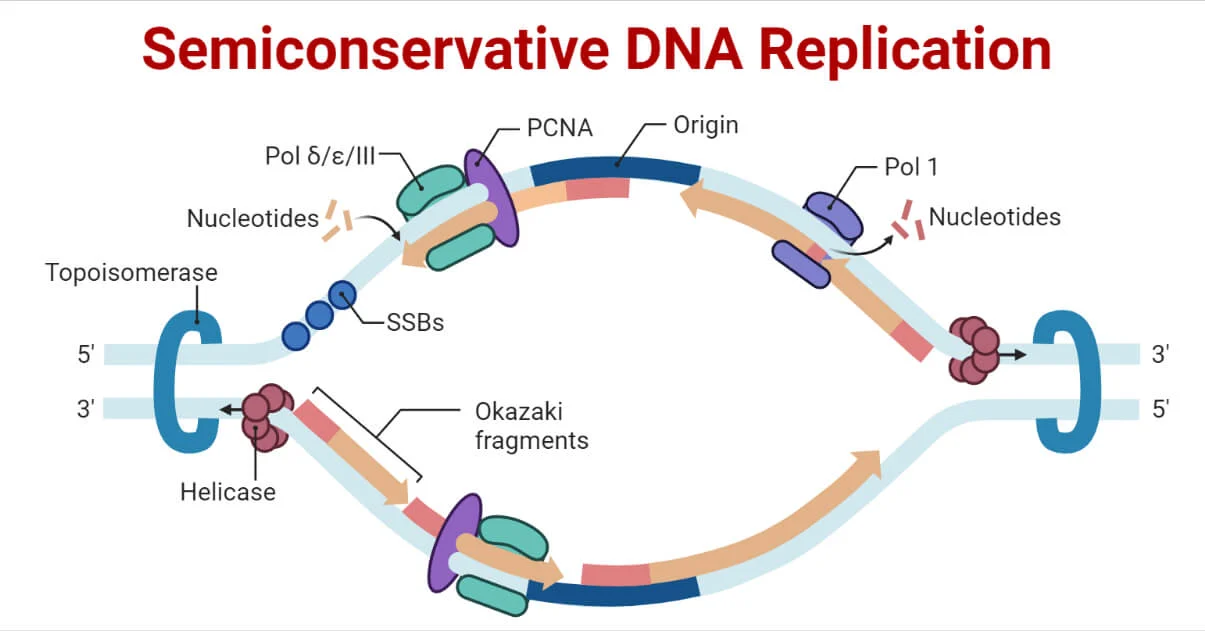
The key points highlighted include:
- Integrated Template System: The double helix structure itself serves as a template for the synthesis of new DNA strands.
- Specificity of Base Pairing: Adenine pairs with Thymine, and Guanine pairs with Cytosine, leading to the automatic determination of the base sequence along each chain.
- Template Function of Double Helix Chains: Each strand of the double helix acts as a template for the synthesis of the complementary strand.
- Replication Process: Watson and Crick proposed a mechanism involving the disruption of hydrogen bonds, rotation, and separation of the two strands.
- Base Attraction and Hydrogen Bonds: Complementary free nucleotides are attracted to each base and held in place by hydrogen bonds.
- Phosphodiester Bond Formation: The linked nucleotides form phosphodiester bonds, creating a new polynucleotide strand.
- Result of Replication: Two identical double-stranded DNA molecules are produced.
- Semi-Conservative Replication: Each progeny retains half of the parent DNA molecule, following a semi-conservative replication pattern.
Additionally, note that while DNA replication is similar in prokaryotes and eukaryotes, there are differences, and the process is more complex in eukaryotes. This complexity in eukaryotic DNA replication arises from the larger size and structural complexity of eukaryotic genomes. The involvement of multiple origins of replication, chromatin structure, and additional regulatory steps are some aspects that contribute to the intricacy of DNA replication in eukaryotes.
Two alternative models of DNA replication
Conservative Replication:
- In this model, the new DNA molecule is composed of two newly synthesized strands.
- Both strands of the parent double helix are entirely conserved without undergoing separation or disruption.
- Essentially, the entire original double helix is maintained intact, and a new, fully synthesized double helix is formed alongside it.
Dispersive Replication:
- Dispersive replication involves the breaking up of the parent double helix during the replication process.
- Fragments of the parent strands are fragmented and dispersed among the newly synthesized strands.
- The result is the formation of two new double helices, each consisting of a mix of original parent fragments and newly synthesized strands.
These models provide alternative hypotheses for the process of DNA replication, suggesting different outcomes in terms of the conservation or dispersal of the original genetic material. It’s worth noting that the semiconservative model, as initially proposed by Watson and Crick, has substantial experimental support, and subsequent research has validated this mode as the predominant mechanism of DNA replication in living organisms.
Enzymes Involved in DNA Replication and Their Functions
Enzyme/protein | Specific Function |
DNA Helicase | It opens the DNA helix by breaking hydrogen bonds between the nitrogenous bases. |
Topoisomerase | It helps relieve the stress on DNA when unwinding by causing breaks and resealing the DNA. |
Single-strand binding (SSB) proteins | Binds to single-stranded DNA to avoid DNA rewinding back. |
Primase | Synthesizes RNA primers needed to start replication. |
DNA pol I | Exonuclease activity removes RNA primer and replaces it with newly synthesized DNA. |
DNA pol II | Repair function |
DNA pol III (α, δ and ε) | Main enzyme that adds nucleotides. |
Sliding Clamp | It helps hold the DNA polymerase in place when adding nucleotides. |
DNA Ligase | Seals the gaps between the Okazaki fragments to create one continuous DNA strand. |
DNA Replication in Prokaryotes:
The process of DNA replication in prokaryotes involves several steps, and it can be summarized into three major stages:
- Initiation:
- Replication begins at the origin of replication, a specific sequence of nucleotides in the DNA.
- Initiator proteins recognize and bind to the origin, leading to the unwinding and separation of the DNA strands.
- Formation of a replication bubble where the replication process initiates bidirectionally.
- Elongation:
- DNA polymerase enzyme adds complementary nucleotides to the template strands.
- Leading strand synthesis occurs continuously in the 5′ to 3′ direction.
- Lagging strand synthesis involves the formation of Okazaki fragments, synthesized in the 3′ to 5′ direction.
- RNA primers are later replaced with DNA, and fragments are joined by DNA ligase.
- Termination:
- Replication proceeds bidirectionally until the entire DNA molecule is copied.
- Termination signals lead to the completion of replication.
- Two daughter DNA molecules are formed, each identical to the original DNA molecule.
In prokaryotes, such as bacteria, the circular nature of the DNA molecule and the presence of a single origin of replication simplify the replication process. The bidirectional replication from a single origin ensures the swift and accurate duplication of the entire genome.
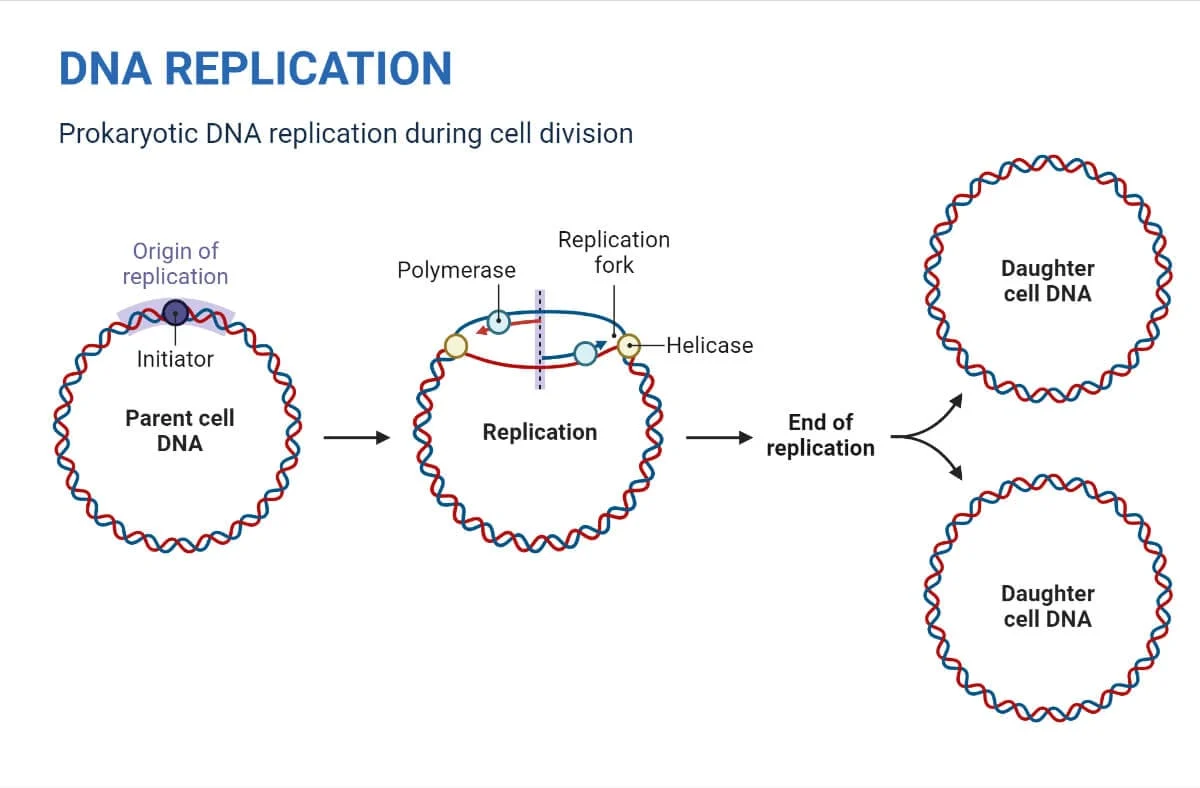
Initiation of DNA Replication in Prokaryotes:
DNA replication in prokaryotes, such as Escherichia coli (E. coli), initiates from a specific origin of replication called OriC. The initiation process involves several key steps:
- OriC Structure:
- OriC is the sole replication origin on the circular bacterial chromosome.
- It is approximately 245 base pairs long and contains several AT-rich sequences.
- Consists of 13 base pair repeats and 9 base pair repeats.
- Binding of DnaA Proteins:
- A cluster of 30 DnaA proteins initially binds to the 9 bp repeats.
- DnaA proteins cause bending of the DNA and open the helix at the 13 bp repeats.
- Loading of DNA Helicase (DnaB):
- DnaC proteins assist DNA helicase (DnaB) in loading onto the origin.
- DNA helicase unwinds the DNA by breaking hydrogen bonds between base pairs, requiring ATP hydrolysis.
- Formation of Replication Forks:
- Y-shaped structures called replication forks are formed as the DNA opens up.
- Two replication forks are established at the origin, proceeding bi-directionally as replication advances.
- Topoisomerase Activity:
- DNA helicase activity induces topological stress, leading to supercoiled DNA.
- Topoisomerase prevents overwinding by temporarily nicking and resealing the DNA.
- Single-Strand Binding (SSB) Proteins:
- SSB proteins coat the single strands near the replication fork.
- Prevents single-stranded DNA from winding back into a double helix.
- RNA Primase and Primer Synthesis:
- DNA polymerase cannot initiate replication and requires a free 3′-OH group.
- RNA primase synthesizes an RNA primer, about 5 to 10 nucleotides long, complementary to the template DNA.
- This primer provides the necessary 3′-OH group for DNA polymerase to initiate nucleotide addition.
The initiation process sets the stage for the subsequent elongation phase of DNA replication. The replication forks continue to grow bidirectionally, and the synthesis of the new DNA strands proceeds in a coordinated manner.
Elongation of DNA Replication in Prokaryotes:
After the initiation phase, the elongation process in DNA replication involves the synthesis of new DNA strands. In prokaryotes, such as Escherichia coli (E. coli), the elongation process proceeds through the following steps:
- Loading of DNA Polymerase III:
- Once priming is complete, DNA polymerase III is loaded onto the DNA template.
- Directionality of Template DNA Strands:
- The two template DNA strands have opposing orientations.
- One strand is in the 5′ to 3′ direction, while the other is oriented in the 3′ to 5′ direction.
- Leading Strand Synthesis:
- The leading strand, complementary to the 3′ to 5′ parental DNA strand, is synthesized continuously.
- It requires only one primer for initiation.
- Lagging Strand Synthesis:
- The lagging strand, complementary to the 5′ to 3′ parental DNA strand, is synthesized in short fragments called Okazaki fragments.
- Multiple primers are required for the initiation of Okazaki fragments.
- Sliding Clamp Protein:
- A ring-shaped protein, the sliding clamp, holds DNA polymerase in place during synthesis.
- Replacement of RNA Primers:
- As synthesis proceeds, RNA primers are replaced by DNA.
- DNA Polymerase I’s Exonuclease Activity:
- DNA Polymerase I removes RNA primers by using DNA behind the RNA as its own primer.
- The gaps left by removing RNA nucleotides are filled with DNA nucleotides.
- Sealing of Gaps by DNA Ligase:
- DNA ligase seals the gaps between the DNA fragments by forming phosphodiester bonds.
- Replication Fork Movement:
- Two replication forks formed at the origin move in opposite directions around the circular chromosome.
- This bidirectional movement ensures the synthesis of both leading and lagging strands.
The coordination of leading and lagging strand synthesis ensures the accurate replication of the entire circular DNA molecule. The DNA replication machinery moves along the template DNA, synthesizing new strands and preserving the genetic information during cell division.
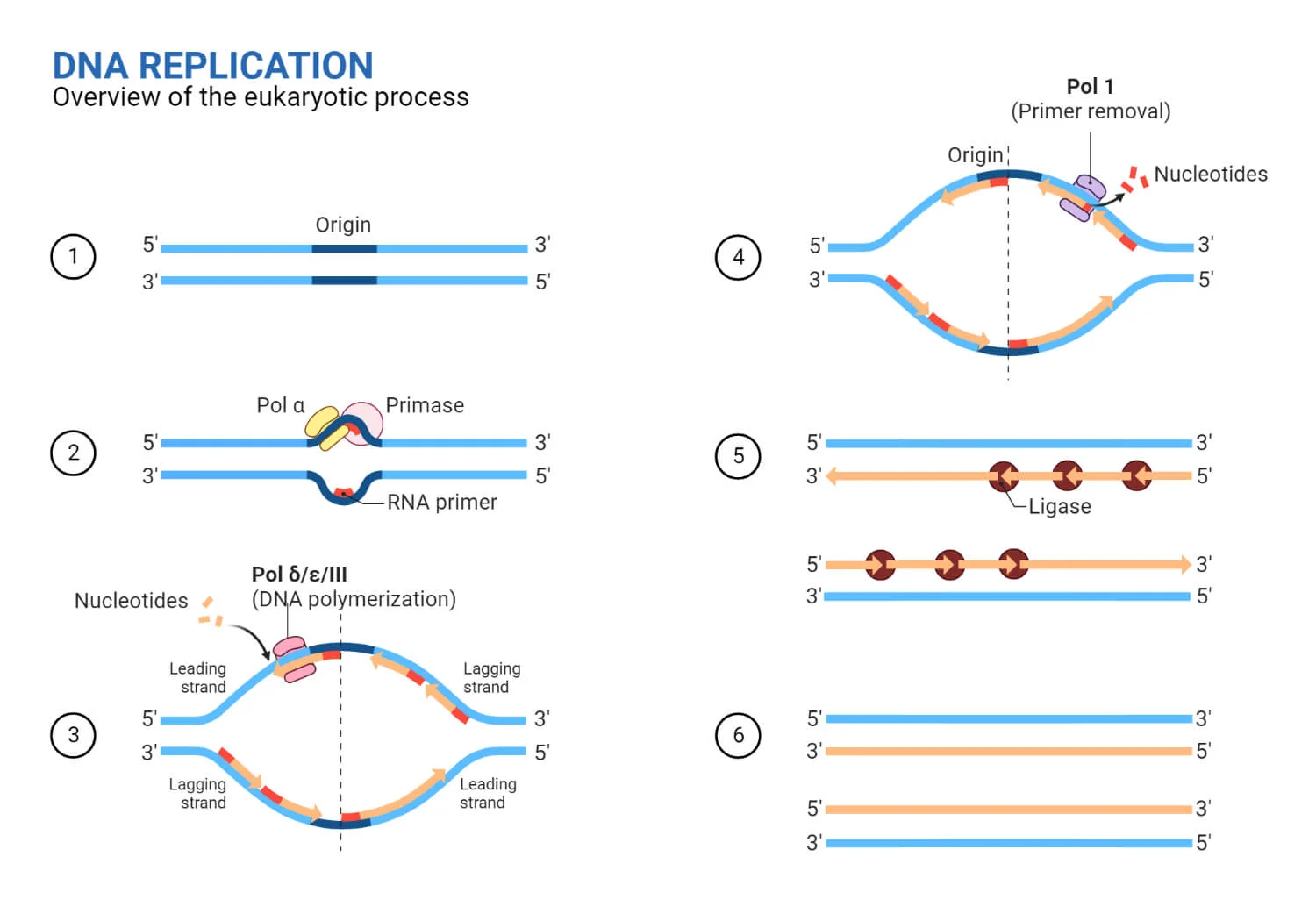
Termination of DNA Replication in Prokaryotes:
The termination phase in DNA replication in prokaryotes marks the completion of the synthesis of new DNA strands. In prokaryotes like Escherichia coli (E. coli), termination involves specific mechanisms:
- Fusion of Replication Forks:
- Termination occurs when the two replication forks, moving in opposite directions, meet and fuse.
- Replication Terminator Protein (Tus) and Termination Recognizing (ter) Sequences:
- Replication terminator protein (Tus) binds to termination recognizing (ter) sequences in the DNA.
- Tus binding blocks replication forks approaching from one direction.
- Halt of Replication Fork Movement:
- The replication fork approaching from one direction is blocked by Tus binding to ter sequences.
- The opposing replication fork halts when it collides with the blocked fork.
- Preventing Tangling of Circular Chromosomes:
- Bacterial circular chromosomes can potentially tangle or even join covalently during replication due to an irregular number of crossovers.
- Role of Topoisomerase IV:
- Topoisomerase IV is involved in decatenating tangled circular chromosomes.
- It resolves any intertwined or linked DNA circles formed during replication.
- Distribution of DNA Copies During Cell Division:
- After termination, the two DNA copies move into two different cells during cell division.
The termination process ensures that each daughter cell receives a complete and accurate copy of the genetic material. It involves the coordination of various proteins, including Tus and topoisomerase IV, to manage the conclusion of DNA replication and the distribution of genetic material during cell division.
Proof-reading of Replicated DNA:
Proof-reading is a crucial mechanism during DNA replication to ensure accuracy in the newly synthesized DNA strands. The process involves scanning the termini of nascent DNA chains for errors and correcting them. Here’s an overview:
- Exonuclease Activity of DNA Polymerase:
- DNA polymerases possess 3′ to 5′ exonuclease activity, allowing them to recognize and correct errors in the newly synthesized DNA.
- Detection and Removal of Mispaired Nucleotides:
- The exonuclease activity scans the 3′ termini of nascent DNA chains.
- When mispaired nucleotides are detected, the exonuclease activity removes them.
- Correct Nucleotide Addition:
- The removal of mispaired nucleotides allows for the addition of the correct nucleotide.
- DNA polymerase’s 5′ to 3′ polymerase activity then adds the correct nucleotide.
- Role of DNA Polymerases in Proofreading:
- In bacteria, all three DNA polymerases (I, II, and III) contribute to proofreading using 3′ to 5′ exonuclease activity.
- Recognition of Incorrect Base Pair:
- When an incorrect base pair is recognized, DNA polymerase reverses its direction by one base pair.
- Base Excision and Correction:
- The polymerase excises the mismatched base, creating an opportunity for correction.
- Following base excision, the polymerase inserts the correct base, allowing replication to continue.
- Impact on Mutation Rate:
- The extent of proofreading in DNA replication directly influences the mutation rate.
- Efficient proofreading minimizes errors, contributing to the fidelity of the genetic information.
Proof-reading mechanisms play a fundamental role in maintaining the integrity and accuracy of the genetic code during DNA replication.
Evidence for Semi-Conservative Replication of DNA in Prokaryotes: Meselson and Stahl Experiment
In 1958, Matthew Meselson and Franklin W. Stahl conducted a groundbreaking experiment that provided compelling evidence for the semi-conservative nature of DNA replication in prokaryotes. Here’s an overview of their experiment:
- Isotopic Labeling:
- Escherichia coli (E. coli) bacteria were cultured for multiple generations in a medium containing NH4Cl with 15N, a heavy isotope of nitrogen.
- Centrifugation Technique:
- The DNA from these cells, labeled with 15N, was isolated and subjected to centrifugation on a salt cesium chloride (CsCl) density gradient.
- Density Separation:
- The DNA separated based on its density, reaching an equilibrium density equal to that of the salt solution.
- Observation of DNA Density:
- The DNA from cells cultured in 15N medium had a higher density than the DNA from cells cultured in standard 14N medium.
- Transfer to 14N Medium:
- E. coli cells with 15N-labeled DNA were transferred to a medium containing 14N and allowed to divide.
- Periodic DNA Extraction:
- DNA was periodically extracted and compared to pure 14N and 15N DNA to observe changes in density.
- Results after One Replication:
- The density of DNA after one replication was found to be intermediate.
- This ruled out conservative replication, which would produce equal amounts of DNA with higher and lower densities but no intermediate density.
- Contradiction with Dispersive Replication:
- Dispersive replication would produce a single density lower than the intermediate density, contrary to the observed results.
- Results after Two Replications:
- DNA from cells after two replications contained equal amounts of DNA with two different densities.
- One density matched the intermediate density of DNA from cells after one division in 14N medium, while the other matched DNA from cells grown exclusively in 14N medium.
- Conclusion:
- The observed pattern was consistent with semi-conservative replication, where double-stranded DNA had one strand of 15N DNA and one of 14N DNA after one replication.
Meselson and Stahl’s experiment provided compelling evidence supporting the semi-conservative model of DNA replication, contributing significantly to our understanding of genetic processes.
DNA Replication in Eukaryotes: Overview of the Process
DNA replication in eukaryotes is a complex and tightly regulated process that ensures accurate duplication of the genetic material. The replication process can be broadly categorized into three major steps:
- Initiation:
- Origin Recognition: Replication begins at specific sites called origins of replication.
- Assembly of Pre-replication Complex (Pre-RC): Origin recognition complex (ORC) binds to origins, facilitating the loading of other initiation proteins.
- Helicase Activation: The MCM helicase complex is loaded onto DNA, forming the pre-RC.
- DNA Unwinding: The pre-RC is activated, leading to the unwinding of DNA at the origin.
- Elongation:
- Primer Synthesis: RNA primase synthesizes short RNA primers complementary to the DNA strands.
- DNA Polymerase Activity: DNA polymerase synthesizes new DNA strands by adding nucleotides complementary to the template.
- Leading and Lagging Strands: DNA synthesis on the leading strand is continuous, while the lagging strand is synthesized discontinuously in Okazaki fragments.
- Ligase Action: DNA ligase joins Okazaki fragments, forming a continuous strand.
- Termination:
- Replication Fork Convergence: Replication forks from adjacent origins converge, leading to the completion of DNA synthesis.
- Decatenation: Enzymes resolve intertwined DNA molecules (catenanes) formed during replication.
- End of Replication: Telomeres at the ends of linear chromosomes pose challenges, and specialized mechanisms ensure their replication and integrity.
Additional Features in Eukaryotic DNA Replication:
- Multiple Origins: Eukaryotic chromosomes have multiple origins to expedite the replication process.
- Cell Cycle Regulation: DNA replication is tightly regulated during the cell cycle, with specific checkpoints ensuring accuracy.
- Telomere Replication: Specialized mechanisms, including telomerase, are involved in replicating the ends of linear chromosomes (telomeres) to counteract the end-replication problem.
The eukaryotic DNA replication process is intricately coordinated and involves the activity of various enzymes, proteins, and regulatory factors. The fidelity of DNA replication is crucial for maintaining genomic integrity and transmitting accurate genetic information to daughter cells during cell division.
Initiation of DNA Replication in Eukaryotes:
The initiation of DNA replication in eukaryotes is a highly regulated and coordinated process involving multiple proteins and complexes. Here is an overview of the key steps in the initiation phase:
- Origins of Replication:
- Eukaryotic chromosomes contain multiple origins of replication. In humans, there can be up to 100,000 origins.
- Each origin serves as a starting point for the replication of a DNA segment.
- Formation of Pre-replication Complex (pre-RC):
- Origin Recognition Complex (ORC): A complex of six ORC proteins (ORC1-6) recognizes and binds to the origin of replication.
- Initiator Proteins: Other initiator proteins, including Cdc6 (Cell division control) and Cdt1 (Chromatin Licensing and DNA Replication Factor), are recruited to form the pre-RC.
- MCM Helicase: Six mini chromosome maintenance (MCM) proteins (MCM2-7) are loaded onto the origin, forming the MCM replicative DNA helicase.
- Activation of the Pre-RC:
- The initiation process begins in the G1 phase of the cell cycle.
- Two kinases, Cyclin-dependent kinase 2 (CdK) and Dbf4-dependent kinase (DdK), initiate the activation of the pre-RC.
- Cdc45 is recruited by CdK and DdK, and this complex then recruits other DNA-replicating proteins.
- Formation of the CMG Helicase:
- Cdc45, MCM2-7, and the GINS complex (Go-Ichi-Ni-San) combine to form the active CMG helicase during the S phase of the cell cycle.
- The CMG helicase unwinds the DNA at the origin, creating the replication fork.
- Replication Fork Formation:
- Y-shaped replication forks are formed as the DNA strands open up at the origin.
- Two replication forks, moving bi-directionally, grow as replication proceeds.
- Topological Changes and Single-Strand Binding:
- Supercoiled DNA forms due to the topological stress caused by DNA helicase activity.
- Replication factor A (RF-A) and Topoisomerase contribute to extensive duplex unwinding by altering the DNA topology at the replication fork.
- Single-strand binding proteins prevent the unwound single strands from re-annealing.
- Primer Synthesis:
- Primase, tightly associated with DNA polymerase α, synthesizes short RNA primers complementary to the DNA strands.
The initiation phase sets the stage for the subsequent elongation and termination phases of DNA replication in eukaryotes. The precise regulation ensures accurate and faithful duplication of the genetic material.
Elongation of DNA Replication in Eukaryotes:
After the initiation complex is formed and cells enter the S phase of the cell cycle, the process of DNA replication enters the elongation phase. The replisome complex coordinates the various activities involved in the synthesis of new DNA strands. Here’s an overview of the key steps in the elongation phase:
- Formation of Replisome:
- The initiation complex transforms into a replisome once the cells progress into the S phase.
- The replisome is a multi-protein complex responsible for coordinating DNA replication.
- Leading Strand Synthesis:
- DNA polymerase ε is responsible for synthesizing the leading strand continuously.
- The leading strand points in the same direction as the DNA unwinding, allowing for continuous synthesis.
- Lagging Strand Synthesis:
- DNA polymerase α initiates the synthesis of short RNA primers (20–30 nucleotides) during the initiation of each Okazaki fragment on the lagging strand.
- DNA polymerase δ then extends the Okazaki fragments on the lagging strand in a fragmented or discontinuous manner.
- Sliding Clamp – PCNA:
- The DNA polymerase is stabilized and held in place by a protein called PCNA (Proliferating Cell Nuclear Antigen), which acts as a sliding clamp.
- PCNA prevents the DNA polymerase from sliding off the DNA template.
- RNA Primer Removal:
- RNase H removes the RNA primers from the Okazaki fragments.
- One ribonucleotide remains attached to the DNA 3′ end of the Okazaki fragment after primer removal.
- Flap Endonuclease 1 (FEN 1):
- FEN 1 removes the remaining ribonucleotide, ensuring the formation of a clean DNA end.
- Gap Filling:
- After primer removal, gaps between Okazaki fragments are filled by DNA polymerase δ, which adds DNA nucleotides to the 3′ ends.
- Nick Sealing – DNA Ligase:
- DNA ligase seals the nick that forms between the Okazaki fragment and the lagging strand.
- This process results in the formation of a continuous, single lagging strand.
The coordination of leading and lagging strand synthesis, along with the precise removal of RNA primers and sealing of nicks, ensures the faithful and accurate replication of the entire DNA molecule. The replisome plays a crucial role in orchestrating these processes during the elongation phase of DNA replication in eukaryotes.
Termination of DNA Replication in Eukaryotes:
The termination phase of DNA replication in eukaryotes involves several key processes that ensure the accurate completion of DNA synthesis and the maintenance of chromosomal integrity. Here is an overview of the termination steps:
- Convergence of Replication Forks:
- Termination occurs when two converging replication forks meet.
- The specific location of termination is not predetermined but depends on the relative start times and progression speeds of the replication forks.
- Torsional Strain and Topoisomerase II:
- As the replication forks converge, torsional strain builds up behind them.
- Topoisomerase II is responsible for managing this torsional strain, ensuring proper winding and unwinding of the DNA.
- Passage of Replicating Complexes:
- The two replisomes (replicating complexes) pass each other in opposite directions.
- It is proposed that the CMG (Cdc45-MCM-GINS) complex slides onto the double-stranded DNA of the last Okazaki fragment.
- Removal of CMG Helicase:
- A crucial step in termination is the removal of the CMG helicase from chromatin.
- CMG helicase removal involves the ubiquitination of MCM7, marking it for dismantling.
- Cdc48 plays a role in disassembling the replisome.
- Linear Chromosomes and Telomeres:
- Eukaryotic chromosomes are linear, and DNA replication does not reach the very end of the chromosome.
- Okazaki fragments, requiring RNA primers ahead of the lagging strand, result in the loss of DNA with each cycle.
- Telomeres and Telomerase:
- Eukaryotic cells address the issue of DNA shortening during replication using telomeres—repetitive nucleotide sequences at the ends of chromosomes.
- Telomeres prevent damage to chromosome ends and are composed of a repetitive sequence like TTAAGGG, repeated 100 to 1000 times.
- Telomerase, an enzyme, prevents cell degradation by extending the telomeric repetitive sequence. This occurs in germ cells, some stem cells, and certain white blood cells.
- Somatic cells experience telomere shortening with each round of replication, leading to the hayflick limit where cells can no longer divide.
- In somatic cells, activation of telomerase is rare, but when it occurs mistakenly, it can contribute to cancer development.
The termination phase ensures the completion of DNA replication with precision, addressing challenges posed by linear chromosomes and the need for maintaining chromosome stability over successive generations.
Proofreading in Eukaryotic DNA Replication:
DNA replication in eukaryotes involves multiple replicative polymerases, each with specific roles and proofreading mechanisms. Here’s an overview of proofreading in eukaryotic DNA replication:
- Replicative Polymerases:
- Three main replicative polymerases are involved in eukaryotic genome replication: Pol α (alpha), Pol δ (delta), and Pol ɛ (epsilon).
- Pol α is responsible for initiating DNA synthesis but has low fidelity due to the absence of internal proofreading.
- Error Rate of Pol α:
- Pol α exhibits a high estimated base substitution error rate of 10^4.
- During each replication cycle, Pol α contributes to the synthesis of approximately 1.5% of the eukaryotic genome.
- It introduces hundreds of mismatches, indicating its lower fidelity.
- Exonucleolytic Proofreading by Pol δ:
- Pol δ, another replicative polymerase, plays a crucial role in correcting errors made by Pol α.
- The exonucleolytic proofreading activity of Pol δ helps maintain higher fidelity during DNA replication.
- Error Correction by Pol δ:
- Errors introduced by Pol δ itself, as well as those induced by Pol α, can be corrected by the proofreading activity of Pol δ.
- Limited Proofreading by Pol ɛ:
- Pol ɛ, while unable to correct errors generated by Pol α or Pol δ, possesses some proofreading capabilities.
- Pol δ can proofread errors introduced by Pol ɛ on the leading DNA strand, contributing to the overall fidelity of DNA replication.
The coordination of these replicative polymerases with proofreading activities helps ensure the accuracy of DNA synthesis during replication. Pol δ, with its exonucleolytic proofreading function, plays a significant role in error correction, contributing to the faithful transmission of genetic information in eukaryotic cells.
Evidence for Semi-Conservative DNA Replication in Eukaryotes:
In 1957, J.H. Taylor and P. Woods conducted experiments using root tip cells of the bean plant (Vicia faba) to provide evidence supporting the semi-conservative replication of DNA in eukaryotes. Their findings were instrumental in confirming the mode of DNA replication. Here’s a summary of the experiment:
- Cell Type and Labeling:
- Root tip cells of Vicia faba were chosen for the experiment.
- The DNA molecules in these cells were labeled with radioactive thymidine (3H).
- Treatment and Label Distribution:
- The labeled root tip cells were treated with radioactive thymidine to ensure that the DNA incorporated the labeled nucleotide.
- Radioactivity (3H label) was evenly distributed in both strands of the DNA.
- Colchicine Addition:
- Colchicine, a substance preventing sister chromatid separation during anaphase, was added to the media.
- This step aimed to maintain the connection between sister chromatids, allowing for the observation of DNA replication patterns.
- Observation of DNA Strands:
- In the first generation, autoradiography and light microscopy were used to observe the labeled DNA strands.
- The original DNA strand with the 3H label was observed alongside another unlabeled DNA strand.
- Only one strand from the second division of DNA exhibited radioactivity.
- Interpretation:
- The even distribution of radioactivity in both strands during the first generation supported the semi-conservative nature of DNA replication.
- The presence of radioactivity in only one strand of the second generation further confirmed the semi-conservative mode, as one strand retained the labeled nucleotides from the original DNA.
Conclusion: The experiment conducted by Taylor and Woods provided strong evidence for the semi-conservative replication of DNA in eukaryotes. The observed distribution of labeled nucleotides in successive generations supported the idea that each newly synthesized DNA molecule consists of one original (labeled) strand and one newly synthesized (unlabeled) strand, confirming the semi-conservative model proposed by Watson and Crick.
Difference between Prokaryotic and Eukaryotic Replication
Property | Prokaryotes | Eukaryotes |
---|---|---|
Origin of replication | Only one origin of replication per molecule of DNA. | Multiple origins of replication in each chromosome. |
Location | Occurs in the cytoplasm. | Occurs inside the nucleus. |
Rate of replication | Replication is rapid (about 1000 nucleotides/s). | Replication is slow (50 to 100 nucleotides/s). |
DNA polymerase types | 5 | 14 |
Telomerase | Not present. | Present. |
RNA primer removal | DNA pol I. | RNase H. |
Strand elongation | DNA pol III. | Pol α, pol δ, pol ε. |
Sliding clamp | Sliding clamp. | PCNA (Proliferating Cell Nuclear Antigen). |