Cellular respiration is a vital biochemical process that occurs in cells to produce energy in the form of adenosine triphosphate (ATP). It involves the breakdown of organic molecules, typically glucose, to release energy. Cellular respiration can be broadly categorized into three main stages: glycolysis, the citric acid cycle (Krebs cycle), and oxidative phosphorylation (electron transport chain and chemiosmosis).
1. Glycolysis:
- Equation: C6H12O6 + 2 ATP + 2 NAD+ → 4 ADP + 4 Pi + 2 NADH + 4 H+ + 2 pyruvate
- Location: Cytoplasm
- Steps: Glycolysis involves the breakdown of one molecule of glucose (6 carbons) into two molecules of pyruvate (3 carbons) through a series of enzymatic reactions.
- Products: 2 ATP (produced), 2 NADH (reduced coenzymes), and 2 pyruvate molecules.
2. Citric Acid Cycle (Krebs Cycle):
- Equation: 2 Acetyl-CoA + 6 NAD+ + 2 FAD + 2 ADP + 2 Pi + 4 H2O → 4 CO2 + 6 NADH + 2 FADH2 + 2 ATP + 4 H+
- Location: Mitochondrial matrix
- Steps: Acetyl-CoA (derived from the breakdown of pyruvate) enters the citric acid cycle, leading to the release of carbon dioxide and the generation of reduced coenzymes (NADH and FADH2).
- Products: 6 NADH, 2 FADH2, 2 ATP, 4 CO2.
3. Oxidative Phosphorylation:
- Equation: 10 NADH + 2 FADH2 + 6 O2 + 34 ADP + 34 Pi → 10 NAD+ + 2 FAD + 34 ATP + 12 H2O
- Location: Inner mitochondrial membrane (electron transport chain and chemiosmosis)
- Steps: Electrons from NADH and FADH2 are transferred through a series of protein complexes in the electron transport chain, leading to the pumping of protons (H+) across the inner mitochondrial membrane. This creates an electrochemical gradient that drives ATP synthesis through chemiosmosis.
- Products: 34 ATP, water (H2O).
Overall, cellular respiration is a catabolic process that produces a net gain of approximately 38 molecules of ATP per molecule of glucose. The process is highly efficient in extracting energy stored in glucose molecules for cellular activities.
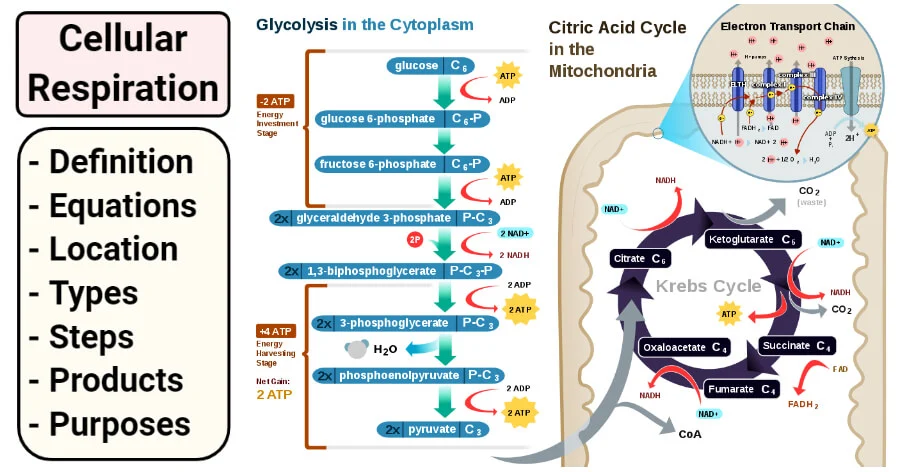
Adenosine Triphosphate (ATP):
- ATP is an organic compound that serves as an energy-carrying molecule in cells.
- It captures and transfers energy produced during chemical reactions within the cell.
- Structurally, ATP is a nucleotide composed of three main units: adenine (a nitrogenous base), ribose (a sugar unit), and three phosphate groups bound to the ribose backbone.
- Unlike carbohydrates and proteins, ATP does not act as a long-term storage unit for energy but rather functions as a shuttle for immediate energy release during cellular activities.
- Energy release occurs through the breaking of phosphate bonds, leading to the formation of adenosine diphosphate (ADP) or adenosine monophosphate (AMP) molecules.
- ATP is not a storage molecule, but rather a dynamic molecule that is constantly being synthesized and hydrolyzed in response to the energy needs of the cell.
- The synthesis of ATP predominantly occurs in the mitochondria, which is often referred to as the powerhouse of the cell due to its role in energy production. However, some ATP can also be produced in the cytoplasm through processes like glycolysis.
Nicotinamide Adenine Dinucleotide (NAD):
- NAD is a coenzyme that plays a crucial role in cellular respiration, serving as a carrier of electrons during various metabolic reactions.
- Structurally, NAD consists of two nucleotide units: one containing adenine as the nucleobase, and the other containing the nicotinamide unit. Two phosphate groups are attached to the nucleotide units.
- NAD exists in two forms based on its oxidation state: NAD+ (oxidized) and NADH (reduced).
- NAD participates in redox reactions, where it becomes reduced by accepting electrons (and a hydrogen ion) and oxidized by donating those electrons to other molecules.
- The interconversion between NAD+ and NADH is a key aspect of the transfer of energy in the form of electrons during cellular respiration.
- NAD can be synthesized in the body from smaller units, including amino acids like tryptophan and aspartic acid.
NAD’s role in redox reactions makes it a vital component in energy-producing processes, such as glycolysis, the citric acid cycle, and oxidative phosphorylation, where it helps shuttle electrons between molecules, facilitating the release of energy.

Flavin Adenine Dinucleotide (FAD):
- FAD is a metabolic coenzyme that serves as an electron carrier in various enzymatic reactions within the body.
- Structurally, FAD is similar to NAD, consisting of two nucleotide units. One unit contains adenine as the nitrogen base, and the other contains flavin units.
- FAD is synthesized in the body from riboflavin (vitamin B2) and two molecules of ATP. The process involves the phosphorylation of riboflavin by ATP, resulting in the formation of flavin mononucleotide (FMN). The transfer of an AMP unit from ATP leads to the formation of FAD.
- In cellular respiration, FAD exists in two oxidation states: FADH (partially reduced) and FADH2 (fully reduced).
- Like NAD, FAD is involved in redox reactions, participating in the transfer of electrons from one molecule to another.
- The multiple oxidation states of FAD allow it to accept and donate electrons, making it a versatile coenzyme in various metabolic pathways, including those related to energy production.
Overall, FAD, along with NAD, plays a crucial role in the electron transport chain during cellular respiration and other metabolic processes, contributing to the generation of ATP and the transfer of energy within the cell.
Types of Cellular Respiration
summary:
Aerobic Respiration Equation:
- In aerobic respiration, which occurs in the presence of oxygen, the overall equation is:
C6H12O6 + 6O2 + 36ADP + 36Pi → 6CO2 + 6H2O + 36ATP - This process is highly efficient and produces a significant amount of ATP.
Anaerobic Respiration Equations:
- In alcoholic fermentation, where oxygen is absent, the overall equation is:
C6H12O6 + 2ADP + 2Pi → 2C2H5OH + 2CO2 + 2ATP - In lactic acid fermentation, also occurring in the absence of oxygen, the overall equation is:
C6H12O6 + 2ADP + 2Pi → 2C3H6O3 + 2ATP - Anaerobic pathways are generally less efficient than aerobic respiration and result in the production of fewer ATP molecules. The specific products and pathways can vary depending on the type of anaerobic respiration (alcoholic fermentation, lactic acid fermentation, etc.).
Anaerobic Respiration:
- Definition: Anaerobic respiration is a type of cellular respiration that occurs in the absence of oxygen, predominantly in prokaryotic organisms.
- Final Electron Acceptor: In anaerobic respiration, other molecules or ions like sulfate or nitrate act as the final electron acceptor, substituting for oxygen.
- Byproduct Variation: The byproduct of anaerobic respiration depends on the specific form of anaerobic respiration being employed.
- Types of Anaerobic Respiration: Anaerobic respiration or fermentation comes in various forms, including alcoholic fermentation, lactic acid fermentation, and methanogenesis, each determined by the electron acceptors and resulting byproducts.
- Alcoholic Fermentation: In alcoholic fermentation, carbohydrates break down to produce alcohol and carbon dioxide.
- Lactic Acid Fermentation: Lactic acid fermentation involves the fermentation of carbohydrates to form lactic acid, carried out by lactic acid bacteria in the absence of oxygen.
- Methanogenesis: Methanogenesis is a unique type of anaerobic respiration resulting in methane and carbon dioxide as byproducts.
- Prevalence: Anaerobic respiration is more common in prokaryotes residing in low-oxygen environments, such as deep-sea surfaces.
- Efficiency Comparison: Anaerobic respiration is less efficient than aerobic respiration, primarily because the final electron acceptor has a smaller reduction potential than oxygen molecules.
- Biogeochemical Importance: Despite its lower efficiency, anaerobic respiration is vital for biogeochemical cycles of elements like sulfur, carbon, and nitrogen.
- Location: The process of anaerobic respiration takes place in the cytoplasm of the cell, as the enzymes required for the process are present in this cellular compartment.
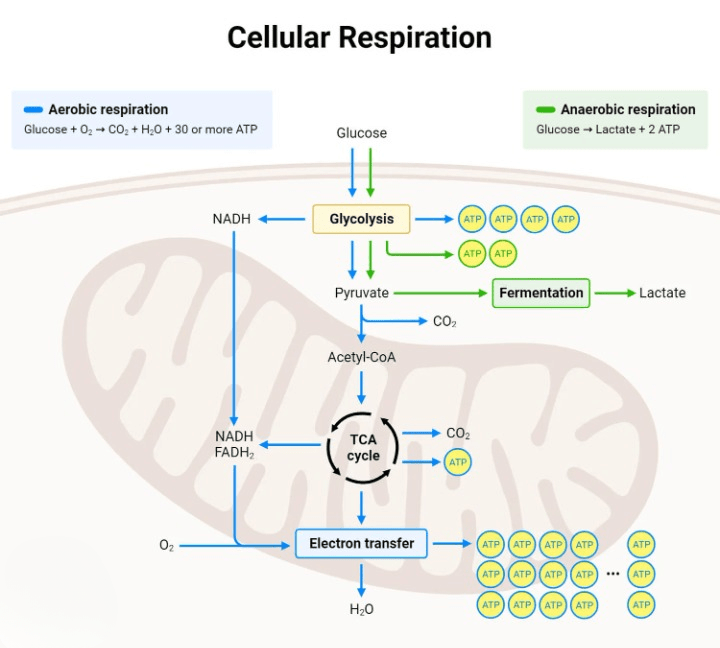
Anaerobic Respiration:
- Definition: Anaerobic respiration is a form of cellular respiration occurring in the absence of oxygen, commonly observed in prokaryotic organisms.
- Final Electron Acceptor: In anaerobic respiration, molecules or ions, such as sulfate or nitrate, substitute oxygen as the final electron acceptor.
- Byproduct Variation: The byproduct of anaerobic respiration varies depending on the specific type of anaerobic pathway employed.
- Types of Anaerobic Respiration: Anaerobic respiration, or fermentation, exhibits diverse forms determined by electron acceptors and resulting byproducts.
- Alcoholic Fermentation: Breaks down carbohydrates to produce alcohol and carbon dioxide.
- Lactic Acid Fermentation: Involves the fermentation of carbohydrates by lactic acid bacteria, resulting in lactic acid, in the absence of oxygen.
- Methanogenesis: A unique anaerobic respiration type producing methane and carbon dioxide as byproducts.
- Prevalence: Anaerobic respiration is more common in prokaryotes residing in environments with limited oxygen, such as deep-sea surfaces.
- Efficiency Comparison: Anaerobic respiration is less efficient than aerobic respiration due to the smaller reduction potential of the final electron acceptor.
- Biogeochemical Importance: Despite lower efficiency, anaerobic respiration plays a crucial role in the biogeochemical cycles of elements like sulfur, carbon, and nitrogen.
- Location: The process of anaerobic respiration takes place in the cytoplasm of the cell, as the required enzymes are present in this cellular compartment.
Cellular Respiration Steps
Glycolysis:
- Definition: Glycolysis is the inaugural step of cellular respiration, breaking down a glucose molecule into pyruvate through a sequence of 10 enzymatic reactions.
- Initiation of Glucose Metabolism: Serving as the initial stage of glucose metabolism, glycolysis is a common pathway in both aerobic and anaerobic respiration.
- Regulation and Pyruvate Fate: The overall sequence of glycolysis reactions may vary among species, impacting the regulation and fate of pyruvate.
- Compound Breakdown: During glycolysis, a six-carbon compound like glucose undergoes breakdown, yielding two three-carbon compounds (pyruvate) and releasing 2 molecules of ATP.
- Relation to Aerobic Respiration: In aerobic respiration, glycolysis acts as a precursor to the citric acid cycle and the electron transport chain, major contributors to ATP production.
- Pathway Choices: The product of glycolysis can proceed along one of three pathways, contingent on the availability of oxygen and metabolic activities.
- Summary Equation:
C6H12O6 + 2ADP + 2Pi + 2NAD+ → 2C3H4O3 + 2H2O + 2ATP + 2NADH + 2H+ - In Words:
Glucose + ADP + Pi + NAD → Pyruvate + Water + ATP + NADH + Hydrogen ions

Pyruvate Oxidation:
- Definition: Pyruvate oxidation is the second step of aerobic respiration, representing one of the possible fates of pyruvate molecules produced in glycolysis.
- Pyruvate Formation: Pyruvate molecules are the end products of glycolysis, a pathway common to both aerobic and anaerobic respiration.
- Fate Determination: The fate of pyruvate is determined by the availability of oxygen and metabolic conditions within the cell.
- Occurrence: Pyruvate oxidation takes place in the presence of oxygen, after the pyruvate molecules are transported from the cytoplasm to the mitochondria.
- Enzyme Catalysis: In this process, pyruvate is dehydrogenated by the enzyme pyruvate dehydrogenase complex, yielding acetyl Co-A and CO2. This enzyme is located in the mitochondrial matrix of eukaryotes and the cytoplasm of prokaryotes.
- Linking Role: Pyruvate oxidation acts as a link between glycolysis and the citric acid cycle in aerobic respiration.
- ATP Production: During pyruvate oxidation, a total of 3 ATP molecules are produced through the entry of NADH into the electron transport chain.
- Overall Reaction: The overall reaction of pyruvate oxidation is summarized as:
Pyruvate Coenzyme A + NAD → Acetyl Co-A + NADH - Location: Pyruvate oxidation occurs in the mitochondria of eukaryotic cells and the cytoplasm of prokaryotic cells.
Citric Acid Cycle:
- Process Description: The citric acid cycle, or Kreb’s cycle, orchestrates the comprehensive oxidation of acetyl CoA, resulting in the release of carbon dioxide and water molecules.
- Metabolic Universality: As the most widespread pathway for aerobic energy-rich molecule metabolism, the cycle’s reactions contribute electrons to the electron transport chain, subsequently reducing oxygen and generating ATP.
- Broad Metabolic Impact: Beyond carbohydrate metabolism, the citric acid cycle plays a crucial role in processing other biomolecules such as amino acids and fatty acids.
- Oxygen Dependency: The cycle necessitates oxygen presence, enabling energy-rich molecules like NAD+ and FAD to recover ATP from their reduced forms through electron transfer to molecular oxygen.
- Dual Purposes: The citric acid cycle serves two main purposes: the disposition of carbon and hydrogen atoms and the conversion of potential chemical energy into metabolic energy, manifested in ATP.
- ATP Production: During the complete oxidation of a single acetyl Co-A molecule, a total of 12 ATPs are formed. However, only one ATP is directly produced by the cycle, with the remaining generated post the entry of high-energy molecules into the electron transport chain.
- Overall Reaction:
CH3CO-SCoA + 3NAD+ + FAD + GDP + Pi + 2H2O → 2CO2 + CoA-SH+ 3NADH + FADH2 + GTP + 2H+

Oxidative Phosphorylation:
- Definition: Oxidative Phosphorylation, also known as the Electron Transport Chain, constitutes the final step of aerobic respiration and involves a series of redox reactions to synthesize ATP molecules.
- Electron Source: Electrons generated in the citric acid cycle are transferred from organic compounds to oxygen, simultaneously releasing energy in the form of ATP.
- Location: Electron transport occurs across four large protein complexes situated in the inner mitochondrial membrane.
- Protein Complexes: The chain comprises proteins with tightly bound prosthetic groups capable of accepting and donating electrons due to their multiple oxidation states.
- ATP Synthesis: The number of ATP molecules synthesized depends on the energy-rich molecule passing down the electrons. For instance, NADH produces 3 moles of ATP, while FADH produces 2 moles of ATP.
- Metabolic Convergence: Oxidative phosphorylation is crucial for the metabolism of all biomolecules, as all metabolic reactions converge at this stage.
- Electron Carriers: Various chemical groups serve as electron carriers during electron transport through the chain. Additionally, four essential enzyme complexes catalyze the transfer.
- Summary Reaction:
NADH + 1/2O2 + H+ + ADP + Pi → NAD+ + ATP + H2O
Lactic Acid Fermentation:
- Fermentation Type: Lactic acid fermentation represents a form of anaerobic respiration where complex organic compounds, such as glucose, are transformed into lactic acid while releasing cellular energy.
- Initiation via Glycolysis: The process commences with glycolysis, which disassembles a single glucose molecule into two pyruvate molecules.
- Oxygen Dependency: Under aerobic conditions, the produced pyruvate undergoes pyruvate oxidation, leading to the formation of carbon dioxide. However, in oxygen-deprived or limited-oxygen scenarios, pyruvate shifts into various fermentation pathways.
- Occurrence and Environment: Lactic acid fermentation takes place in anaerobic organisms inhabiting low-oxygen environments or in muscle cells during strenuous exercise.
- Enzymatic Catalyst: Lactic dehydrogenase is the pivotal enzyme driving lactic acid fermentation. It facilitates the conversion of pyruvate to lactic acid while oxidizing NADH to NAD+.
- Energy Yield: NAD+ generated in lactic acid fermentation contributes to the production of 2 ATPs through the electron transport chain.
- Metabolic Recycling: Lactic acid accumulated in muscle cells is transported to the liver, where it is reconverted into pyruvate for subsequent utilization in aerobic respiration.
- Industrial and Culinary Significance: Beyond its metabolic role, lactic acid fermentation finds utility in the cost-effective processing and preservation of vegetables, offering diverse and palatable flavors. Industrially, lactic acid fermenting bacteria play a crucial role in the production of dairy products like yogurt and cheese.
Alcoholic Fermentation:
- Definition: Alcoholic fermentation is a form of anaerobic respiration where a carbohydrate molecule undergoes partial oxidation to produce alcohol as a byproduct.
- Pyruvate Conversion: The conversion of pyruvate to ethyl alcohol involves a two-step process, comprising an oxidation reaction followed by a reduction reaction.
- Commonality with Other Pathways: The initial step of alcoholic fermentation mirrors that of aerobic respiration and lactic acid fermentation. Pyruvate serves as the substrate, as in lactic acid fermentation.
- Decarboxylation: In the first step, pyruvate molecules produced from glycolysis undergo decarboxylation catalyzed by pyruvate decarboxylase, yielding acetaldehyde.
- Reduction to Ethanol: Acetaldehyde is further reduced to ethanol by NADH in the presence of alcohol dehydrogenase enzyme.
- NADH Oxidation: NADH is oxidized to form NAD+, producing ATP through the electron transport chain.
- End Products: The final products of alcoholic fermentation are ethanol and carbon dioxide.
- Industrial Importance: The production of alcohol from carbohydrates is significant in industries involved in producing alcoholic beverages like beer and wine.
- Microorganism Utilization: Alcoholic fermentation serves as the primary mode of cellular respiration in yeasts and various other microorganisms.
- Caution: While alcoholic fermentation is advantageous in various applications, the accumulation of large quantities of alcohol can be detrimental to certain microorganisms.
Methanogenesis:
- Definition: Methanogenesis is a distinctive form of anaerobic respiration characterized by the breakdown of carbohydrate molecules into methane and carbon dioxide as byproducts.
- Organisms and Domain: Organisms utilizing methanogenesis are primarily found in the domain Archaea, often in close association with anaerobic bacteria.
- Metabolic Significance: Methanogenesis holds significant metabolic importance as it represents the final step in the decomposition of biomass.
- Anaerobic Nature: Being an anaerobic process, methanogenesis relies on a carbon compound as the terminal electron acceptor. Common electron acceptors in this process include acetic acid or carbon dioxide.
- Biological Decomposition: Methanogenesis plays a crucial role in the decay of organic matter. During advanced stages of biodecomposition, when other electron acceptors are depleted, carbon dioxide becomes the primary electron acceptor in methanogenesis.
- Symbiotic Relationships: Some symbiotic bacteria, residing in the digestive tracts of ruminants, perform methanogenesis. This symbiotic relationship aids in the digestion of organic matter that would otherwise be challenging for the cattle to degrade.
Products of Cellular Respiration:
1. ATP (Adenosine Triphosphate):
- Definition: ATP is the primary and crucial product of cellular respiration, serving as a carrier molecule for storing and transporting energy.
- Energy Storage: ATP stores energy in phosphate bonds, releasing it upon bond breakage as needed.
- Conversion to ADP: After energy release, ATP is converted into ADP (Adenosine Diphosphate).
- Reformation of ATP: ADP molecules regain energy to reform ATP, allowing for the continuous recycling of this energy carrier.
- Efficiency Determination: The efficiency of cellular respiration is assessed by the total number of ATP molecules produced. In aerobic respiration, around 36 ATPs are generated, while anaerobic respiration yields a lower number.
2. Carbon Dioxide:
- Formation: Carbon dioxide is a universal byproduct of cellular respiration, generated as a waste product.
- Toxicity Considerations: Accumulation of high levels of carbon dioxide may induce toxicity by increasing the pH of the cytoplasm. Interaction with water can lead to the formation of carbonic acid, potentially harmful to cells.
- Active Removal: The removal of carbon dioxide from the cell is an active process, requiring energy.
3. Other Products:
- Dependence on Respiration Type: The additional products formed during cellular respiration vary based on the specific type of respiration.
- Anaerobic Respiration Products: In anaerobic respiration or fermentation, common products include acetic acid, ethyl alcohol, methane, lactic acid, propionic acid, etc.
- Aerobic Respiration: Water molecules are produced during aerobic respiration after oxygen molecules capture electrons.
Purpose of Cellular Respiration:
- Energy Production:
- Primary Objective: The fundamental purpose of cellular respiration is to generate the energy required for diverse cellular activities.
- ATP Production: Cellular respiration results in the production of ATP (Adenosine Triphosphate), serving as a crucial energy carrier.
- Energy Utilization: ATP is utilized in various cellular processes such as biosynthesis, locomotion, and active transport.
- Biosynthesis of Biomolecules:
- Intermediate Products: The end and intermediate products of cellular respiration are utilized in the biosynthesis of various biomolecules.
- Building Blocks: These products serve as essential building blocks for the synthesis of carbohydrates, lipids, proteins, and nucleic acids.
- Carbon Cycle Participation:
- Carbon Recycling: Cellular respiration is integral to the carbon cycle, acting as a natural waste management system.
- Carbon Dioxide Release: During respiration, carbon dioxide is released, contributing to the carbon cycle by being absorbed by plants during photosynthesis.
- Metabolism of Biomolecules:
- Amino Acids and Fatty Acids: The steps involved in cellular respiration are crucial for the metabolism of biomolecules such as amino acids and fatty acids.
- Industrial Applications:
- Anaerobic Respiration Processes: Industrial significance is found in anaerobic respiration processes like alcoholic fermentation and lactic acid fermentation.
- Commercial Product Production: These processes are employed to produce various commercial products, contributing to industries such as food and beverage production.
In summary, cellular respiration plays a central role in providing energy, contributing to biosynthesis, participating in the carbon cycle, supporting biomolecule metabolism, and offering industrial applications.