Transcriptomics is a branch of molecular biology that focuses on studying the transcriptome of cells, which consists of all the RNA molecules transcribed from the genome. This includes messenger RNA (mRNA) as well as non-coding RNAs such as ribosomal RNA (rRNA), transfer RNA (tRNA), and microRNAs (miRNAs). By studying RNA, transcriptomics provides insights into gene expression, regulation, and how cells respond to environmental or internal signals.
Transcriptomics is crucial for understanding how genetic information is translated into cellular functions and how gene expression varies in different conditions, tissues, or organisms. High-throughput techniques like microarrays and RNA sequencing (RNA-Seq) allow scientists to analyze RNA at a large scale, facilitating deeper insights into biology and disease mechanisms.

Historical Development of Transcriptomics:
- Early Techniques: Before transcriptomics emerged as a field, RNA was studied using labor-intensive methods such as Northern blotting and Sanger sequencing, which could only analyze a limited number of genes.
- 1990s: The term “transcriptome” was coined in the early 1990s, and the first attempts to study whole transcriptomes began in 1991 with a partial transcriptome from the human brain.
- Expressed Sequence Tags (ESTs): Introduced in the 1990s, ESTs allowed researchers to sequence short parts of cDNA from expressed genes and create gene expression libraries.
- Serial Analysis of Gene Expression (SAGE): Developed in the mid-1990s, this technique allowed high-throughput sequencing of the transcriptome, identifying thousands of gene expressions simultaneously.
- Microarrays: In the mid-1990s, microarrays emerged as a popular technology for studying gene expression. This method enabled the study of thousands of genes on a single chip.
- RNA-Seq: Introduced in the mid-2000s, RNA-Seq revolutionized transcriptomics by providing high-throughput sequencing of entire RNA transcripts, allowing for a more detailed and accurate analysis of gene expression.
Types of Transcriptomics:
Different types of transcriptomics allow researchers to study gene expression at various levels and in different contexts. The major types include:
Bulk Transcriptomics:
- Description: Involves studying the gene expression of bulk RNA samples, typically obtained from a population of thousands or millions of cells.
- Method: RNA is extracted, and techniques like RNA-Seq or microarrays are used to study the gene expression profile of the entire sample.
- Advantages:
- Large-scale gene expression analysis.
- Useful for identifying overall gene expression patterns in a particular condition or tissue.
- Limitations:
- Does not provide insights into the diversity of individual cells in a heterogeneous population.
- Rare cell types or populations may be overlooked.
Single-Cell Transcriptomics:
- Description: Studies gene expression at the level of individual cells, allowing for the exploration of cellular diversity and rare cell types.
- Method: Single-cell RNA sequencing (scRNA-Seq) captures gene expression profiles from individual cells within a sample.
- Advantages:
- Provides detailed information on the transcriptional activity of individual cells.
- Enables the discovery of rare or novel cell types that may be hidden in bulk transcriptomics data.
- Applications:
- Studying cellular heterogeneity.
- Identifying specific cell types in complex tissues like tumors or the brain.
Spatial Transcriptomics:
- Description: Combines transcriptomic analysis with spatial data to study gene expression within the context of tissue architecture.
- Method: Spatially resolved RNA sequencing or imaging-based technologies are used to capture gene expression while maintaining spatial information.
- Advantages:
- Allows the study of gene expression along with the physical location of cells in tissue.
- Preserves the cellular organization and interactions within the tissue.
- Applications:
- Studying tissue development and disease progression (e.g., cancer metastasis).
- Mapping the expression of genes in organs or regions of the brain.
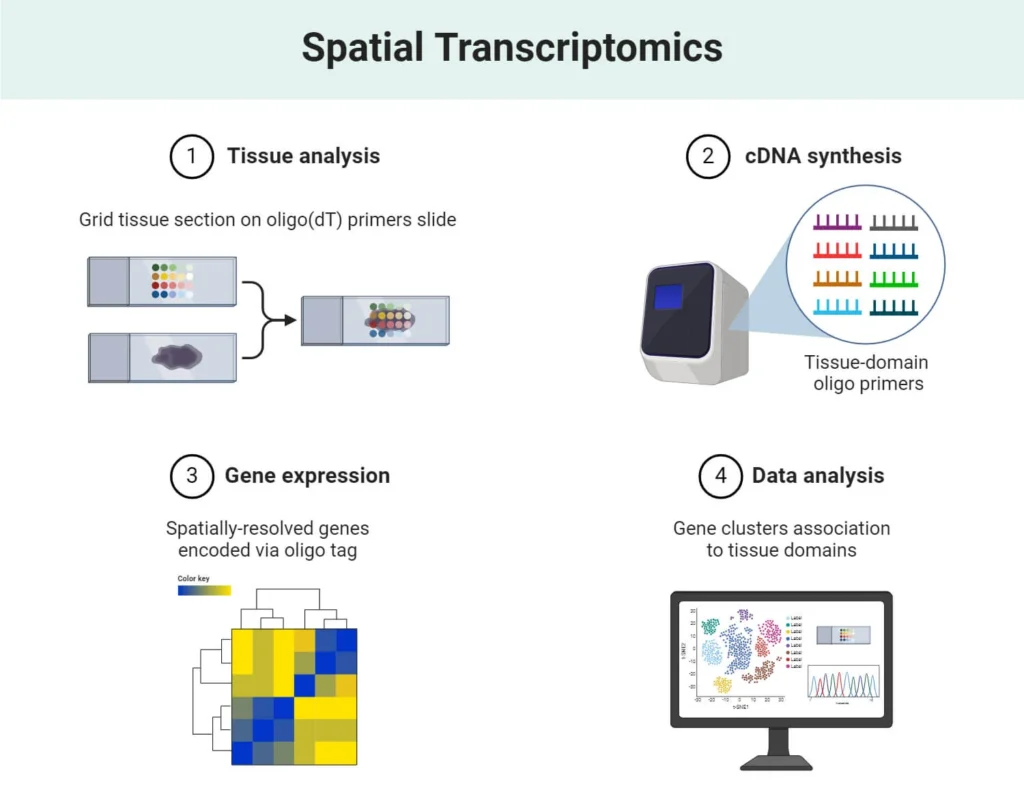
Meta Transcriptomics:
- Description: Studies the collective gene expression of communities of organisms, such as microbial ecosystems, across different environments.
- Method: RNA is extracted from environmental samples, including soil, water, or the human microbiome, and sequenced to profile the gene expression of various organisms.
- Advantages:
- Provides insights into the functional roles of microbial communities in different ecosystems.
- Useful for understanding complex interactions in microbiomes.
- Applications:
- Environmental studies, including soil and water microbiomes.
- Studying the human gut microbiome and its role in health and disease.
Methods Used in Transcriptomics:
Transcriptomics involves several techniques to measure RNA and gene expression. The following are key methods:
Expressed Sequence Tag (EST) Sequencing:
- Method: RNA is converted into complementary DNA (cDNA), which is then sequenced to identify gene expression.
- Role: ESTs were crucial in early transcriptomics and contributed to the development of gene expression libraries.
- Current Relevance: ESTs are now less commonly used in favor of high-throughput RNA-Seq methods but were important in the early stages of transcriptomics.
Serial Analysis of Gene Expression (SAGE):
- Method: SAGE involves generating short sequence tags from mRNA, which are then sequenced to quantify gene expression levels.
- Advantages:
- Provides a quantitative profile of gene expression.
- Helps in identifying novel genes.
- Limitations:
- Short sequence tags may lead to difficulties in identifying unknown genes.
- Issues with tag redundancy and overlap in sequences.
Microarrays:
- Method: Microarrays are used to measure gene expression by hybridizing labeled cDNA to probes on a solid surface (e.g., glass slide).
- Advantages:
- Cost-effective for large-scale gene expression studies.
- Easier to handle small datasets compared to RNA-Seq.
- Limitations:
- Less sensitive to low-abundance transcripts.
- Requires prior knowledge of the genes being studied, making it less effective for discovering new transcripts.

RNA Sequencing (RNA-Seq):
- Method: RNA is converted into cDNA, which is then sequenced using high-throughput next-generation sequencing (NGS) platforms.
- Advantages:
- Provides a comprehensive and unbiased view of the transcriptome.
- Can detect both known and novel transcripts.
- Sensitive enough to detect low-abundance transcripts.
- Challenges:
- RNA-Seq is relatively expensive and requires advanced computational resources for data analysis.
- Data analysis can be complex due to the large volumes of information generated.
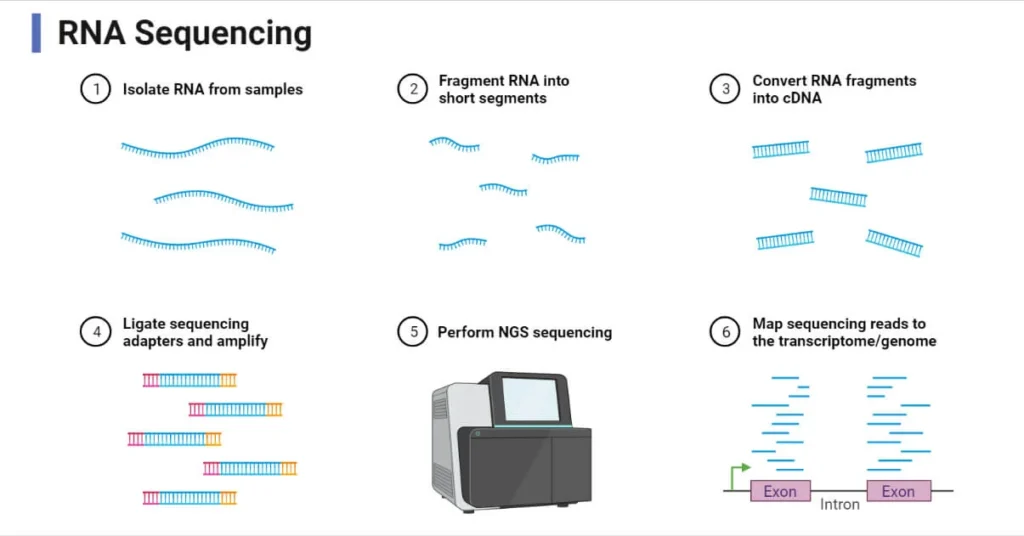
Applications of Transcriptomics:
Transcriptomics has a wide range of applications across various fields of biology and medicine:
- Disease Diagnosis and Profiling:
- Cancer: RNA-Seq can be used to profile gene expression in tumors and identify biomarkers for diagnosis and prognosis.
- Neurodegenerative Diseases: Studies have used transcriptomics to investigate gene expression changes in diseases like Alzheimer’s and Parkinson’s.
- Pharmacogenomics:
- Personalized Medicine: Transcriptomics can help understand how individuals respond to specific drugs based on their gene expression profiles, aiding in personalized treatment strategies.
- Drug Development: By identifying gene expression changes associated with drug responses, transcriptomics can assist in developing new therapeutic drugs.
- Immunology and Host-Pathogen Interactions:
- Immune Responses: Transcriptomics helps to study how immune cells respond to infections or vaccines.
- Pathogen Identification: It can identify gene expression changes in host organisms during pathogen infection.
- Developmental Biology:
- Gene Expression Across Development: Transcriptomics helps study how gene expression changes at different stages of an organism’s development, from embryo to adult.
- Stem Cell Research: Transcriptomics is useful in understanding stem cell differentiation and identifying factors that regulate development.
- Noncoding RNAs:
- Regulatory Roles: Noncoding RNAs, such as microRNAs and long noncoding RNAs, play critical roles in gene regulation. Transcriptomics allows researchers to explore their functions in various cellular processes.
Transcriptomics has become an essential tool for understanding gene expression and regulation at a genome-wide level. Advances in sequencing technologies like RNA-Seq and the development of methods such as single-cell and spatial transcriptomics have greatly expanded our ability to study gene expression in greater detail. With applications ranging from disease diagnosis and drug development to the study of microbial communities, transcriptomics is poised to continue making a significant impact on biological and medical research.